Demonstration of Long-Term Culture and In-Plate Staining Protocols Using the Cellasic® ONIX Microfluidic Platform
Introduction
The ability to analyze cell responses to perturbations over time greatly extends the precision and biological relevance of in vitro studies1. However, there are technical challenges to successful long-term cell culture and analysis. Not only is it difficult to control the temperature and gas composition of the cell environment during the course of the experiment without impeding optical access to the cells, but it is also challenging to maintain sufficient nutrient supply and outflow of waste to keep cells healthy.
The easy-to- use CellASIC® ONIX Microfluidic Platform delivers precise control of perfusion, temperature and gas conditions, facilitating long-term cell culture and enabling automated, in-plate cytochemical analysis using minimal reagent volumes.
Here, we demonstrate the long-term culture of multiple cell lines using autaomated perfusion protocols set up with intuitive CellASIC® ONIX FG Software, comparing the results to those obtained using traditional chambered slides. Our results indicate that optimal experimental parameters, such as flow rates, flow duration, extracellular matrix, gas composition and medium change intervals, can vary greatly with respect to the particular cells being cultured. We also provide step-by- step guides for live/dead staining of cells and automated immunostaining of cells within the microfluidic plate, showing that not only do these automated protocols offer significant time savings, but also that the use of low-volume microfluidics can conserve precious antibodies and staining reagents.
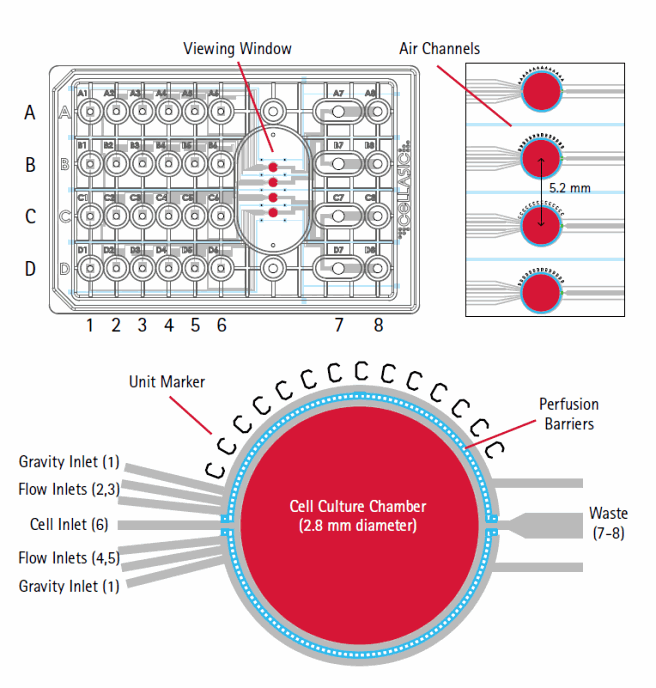
Figure 1.The M04S plate contains four independent flow units (A-D), each with four upstream solution inlets, a gravity flow inlet, a cell inlet, and two waste wells. The culture chamber is 2.8 mm in diameter (120 µm height) and is surrounded with a microfabricated perfusion barrier. Inlet 1 is a gravity flow well, allowing long-term cell culture in a standard incubator without a pressure system. Continuous flow of solutions from inlets 2-5 can create a dynamic exposure profile for viewing responses in live cells.
The CellASIC® ONIX Microfluidic System, in conjunction with the M04S microfluidic plate, provides perfusionbased microenvironment control for long-term, high quality, live cell analysis (Figure 1, previous page). The microfluidic chamber recreates the physiologic mass transport condition for optimized cell health. Four upstream fluidic channels allow controlled exposure of the cells to different solutions during live analysis. The plate can also be cultured in a standard incubator using a dedicated gravity driven flow channel. The cells are in contact with a #1.5 thickness (170 µm) optical glass surface, enabling high quality visualization using an inverted microscope. An integrated microincubator system delivers temperature and gas control to the microfluidic chambers.
Materials and Methods
We used the CellASIC® ONIX Microfluidic Platform for the long-term perfusion of three cell lines, NIH 3T3, MCF7 and PC-3. The CellASIC® ONIX Microfluidic System (Catalogue No. EV262) delivered a controlled perfusion of the cells while the CellASIC® ONIX Microincubator Controller (Catalogue No. MIC230) provided the microenvironmental conditions required for healthy cell culture. The assembled CellASIC® ONIX M04S Microfluidic Plate and manifold with recirculating heater (Catalogue No. F84-HG3) were kept on the microscope stage and this continuous viewing of the live cell culture. Cell proliferation was monitored and compared to a control culture in the incubator.
Cells, media and reagents
NIH 3T3 cells (ATCC®, Catalogue No. CRL-1658) were cultured in EmbryoMax® Dulbecco’s Modified Eagle’s Medium (DMEM, Catalogue No. SLM-220- B), 10% EmbryoMax® fetal bovine serum (FBS, Catalogue No. ES-009- B), 1% EmbryoMax® L-glutamine (Catalogue No. TMS- 002-C), and 1% EmbryoMax® penicillin-streptomycin (Catalogue No. TMS-AB2- C).
MCF7 cells (ATCC® Catalogue No. HTB-22) were cultured in Eagle’s Modified Essential Medium (EMEM, ATCC® Catalogue No. 30-2003) with 10% EmbryoMax® FBS, 0.01 mg/ mL bovine insulin (Sigma, Catalogue No. I0516), and 1% EmbryoMax® penicillin-streptomycin.
PC-3 cells (ATCC® Catalogue No. CRL-1435) were cultured in F-12K medium (ATCC® Catalogue No. 30-2004) with 10% EmbryoMax® FBS and 1% EmbryoMax® penicillin-streptomycin.
Initial cell counting and viability determination
For experiments, cells were trypsinized, harvested by centrifugation, and resuspended in complete medium. 50 µL of each cell sample was counted using the Scepter™ 2.0 handheld automated cell counter (Catalogue No. PHCC20060).
Loading of cells into M04S plate
All four cell chambers of M04S plate were primed with media containing 10% FBS (for NIH 3T3 cells) or with 0.1% gelatin (for MCF7 cells and PC-3 cells) via capillary action prior to cell loading. While the plate was being primed, the temperature calibration plate was sealed to the CellASIC® ONIX manifold and calibrated to ensure that the experiment would take place at the desired temperature (37 °C). 10 µL of cell suspension was added to each cell inlet (well 6).
Programming automated perfusion culture
The intuitive graphical user interface of the CellASIC® ONIX FG software was used to create automated protocols (Table 1) that maintained healthy long-term cultures of NIH 3T3, MCF7 and PC-3 cells in a CellASIC® ONIX M04S plate, on the microscope stage. In the software, the "M04S" tab was selected. After clicking the "Create Protocol" tab, boxes were checked (as shown in the example in Figure 2) to define the flow source, flow rate and flow duration for each step of the protocol.
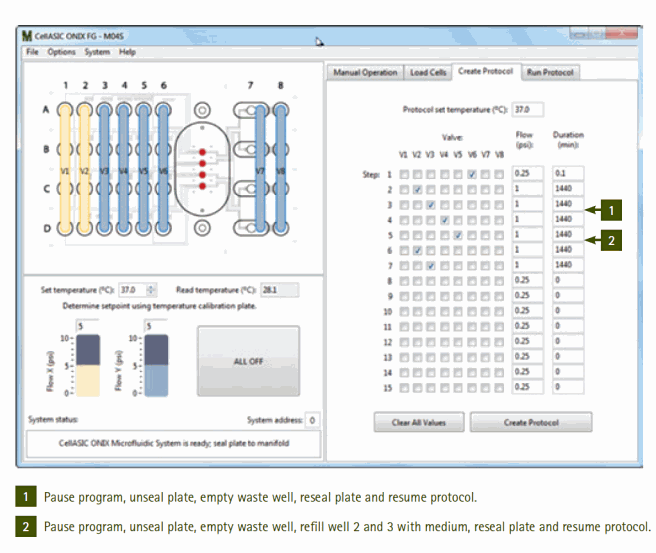
Figure 2. Software interface for creating automated protocol for pressuredriven cell loading followed by long-term perfusion culture (for NIH 3T3 cells). Protocol steps were defined by checking the boxes corresponding to the correct inlet well valve and indicating desired flow rate and duration. At two points in the experiment (shown by arrows), the program was paused and excess media were removed from outlet wells. If needed, media in solution inlet wells were replenished before resealing the M04S plate to manifold.
Cells and media were preloaded into the cell inlet and solution inlet wells, respectively, of a M04S plate. An uninterrupted protocol was created in which inlet well 6 was set to flow at 0.25 psi for 0.1 min (Step 1), for pressure-driven loading of cells. To achieve continuous perfusion, each solution inlet well (wells 2-5) was filled with 300 µL medium, allowing scheduled medium flow from different solution inlets throughout the experiment. Flow rates for inlet wells 2-5 were set to 1 psi for NIH 3T3 cells and 0.25 psi for MCF7 and PC-3 cells. The M04S plate was sealed to the CellASIC® ONIX manifold, which connected the plate to the CellASIC® ONIX Microfluidic System and CellASIC® ONIX Microincubator Controller. The recirculating heater was set to 37 °C. The plate was placed on the microscope stage to visualize cell loading and to monitor long-term cell culture outside of the incubator.
Images were acquired during perfusion at periodic intervals. Also, the program was paused at two or three points and excess medium was removed from outlet wells. If needed, medium in solution inlet wells was replenished before resealing the M04S plate to the manifold.
Preparation for live/dead staining of MCF7 cells within microfluidic plate
The LIVE/DEAD® Viability Kit (Life Technologies) was prepared according to the manufacturer’s protocol. In this assay, live cells, stained with Calcein-AM, fluoresce green, and dead cells, stained with EthD-1, fluoresce red. The M04S microfluidic plate was unsealed from the manifold and temperature set to RT. The wells to be used for live/dead staining were aspirated. 300 µL of live/ dead stain was added to wells A2, C2, A4 and C4. 300 µL of phosphate-buffered saline (PBS, Catalogue No. BSS-1006- A) was added to wells A3, C3, A5 and C5. The manifold gasket was wiped with 70% ethanol. The plate was then sealed to the manifold and the CellASIC® ONIX FG Software was used to create and run the automated staining protocol.
Automated, in-plate viability staining protocol for MCF7 cells after 168-hour perfusion
The M04S plate, prepared for live/dead staining, was sealed to the manifold and placed on the lab bench and a two-step protocol was created in order to flow live/dead stain, followed by PBS, into the cell chambers:
1. Step 1 (live/dead stain): Valves V2 and V4, pressure = 0.5 psi, 60 min
2. Step 2 (PBS): Valves V3 and V5, pressure = 0.5 psi, 5 min
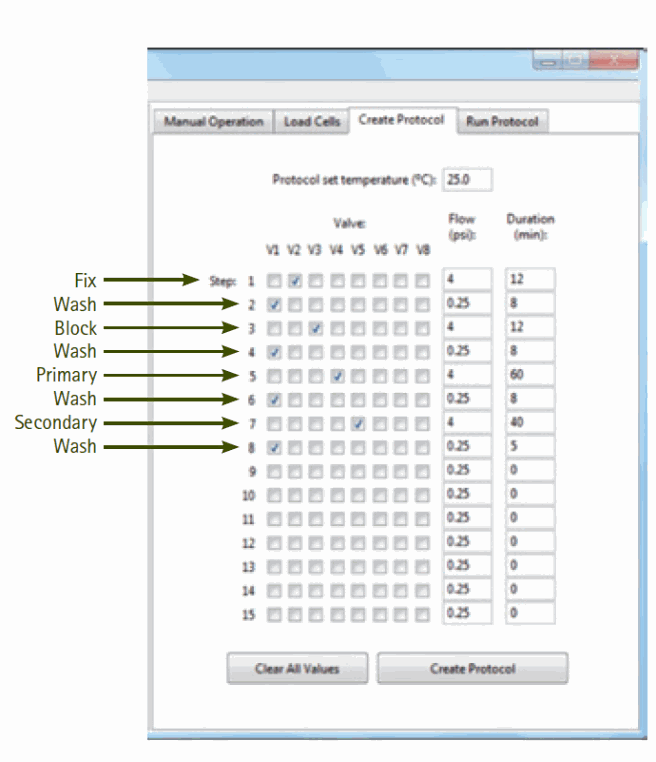
Figure 3. Automated immunostaining protocol for PC-3 cells set up on the CellASIC® ONIX Microfluidic Platform. This screenshot of the automated protocol shows the flow rates and flow duration for each staining step. Refer to Table 2 for a list of solutions used to fill each inlet well.
Automated immunostaining of PC-3 cells
The manifold was set to room temperature and immunostaining solutions were prepared. Staining materials included 4% paraformaldehyde, Triton® X-100 (Sigma), bovine serum albumin (BSA), PBS, mouse anti-cytokeratin 19 (Catalogue No. MAB3238), anti-CD9 (Catalogue No. CBL162), Alexa Fluor® 488 Donkey Anti- Mouse Antibody (Life Technologies) and Hoechst 33342 (Life Technologies). Inlet wells used for immunostaining were aspirated and then filled as shown in Table 2. The manifold gasket was wiped with 70% ethanol. The plate was then sealed to the manifold and the CellASIC® ONIX FG Software was used to create and run the automated staining protocol (Figure 3).
Culture of NIH 3T3 cells in the Millicell® EZ SLIDE
5500 NIH/3T3 cells in 500 µL of medium were added to well 5 of a Millicell® EZ SLIDE (Catalogue No. PEZGS0896). Cells were then incubated at 37 °C for 144 hours. Cells were removed from the incubator for visualization (24 h, 72 h, 144 h) and medium was exchanged every 48 hours.
Culture of MCF7 cells in the Millicell® EZ SLIDE
1.9 x 10 4 MCF7 cells in 500 µL of medium were added to the wells of a Millicell® EZ SLIDE. Cells were then incubated at 37 °C for 168 hours. Cells were removed from the incubator for viewing after 24, 96 and 168 h, and medium was exchanged every 48 hours.
MCF7 cell viability staining after 168 hour culture in the Millicell® EZ SLIDE
Each well was filled with 300 µL of LIVE/DEAD® stain and cells were incubated at room temperature for 60 minutes. After the staining step, wells were aspirated and filled with 300 µL of PBS and incubated at room temperature for 5 minutes before visualization.
Culture of PC-3 cells in Millicell® EZ SLIDE
1.7 x 10 4 PC-3 cells in 500 µL of medium were added to the wells of Millicell® EZ SLIDE. Cellswere then incubated at 37 °C for 144 hours. Cells were removed from the incubator for visualization at 24, 72 and 144 h and medium was exchanged every 48 hours.
Microscopy
All images were acquired with an Olympus® Q Color 5 Camera attached to a Zeiss Axiovert® inverted microscope, using ImagePro software. Scale bars are equal to 100 µm. ImageJ software was used in cases requiring merging of images.
Results
Equal, even pressure-driven cell loading into chambers of microfluidic plates
Making biologically relevant conclusions by comparing one cell culture chamber to another in a single multichamber plate is only possible if cells are evenly loaded and subject to the same perfusion conditions in space and time. The CellASIC® ONIX Microfluidic Platform provides options for pressure-driven loading, as described here, or capillary-driven loading, which allows the user to simply load cells using a pipette in any sterile laminar flow hood without any external systems 2 . We loaded NIH 3T3 cells (at 1.0 x 10 6 cells/mL), MCF7 cells (at both 1.0 x 10 6 and 2.0 x 10 6 cells/mL) and PC-3 cells (at both 1.0 x 10 6 and 2.0 x 10 6 cells/mL) into replicate chambers of the CellASIC® ONIX M04S Microfluidic Plate. The resulting even distribution of the cells, not only across each chamber surface, but also when comparing each chamber to the next, illustrated the precision with which the user can control the flow from the inlet wells of the CellASIC® ONIX Microfluidic System (Figure 4 shows MCF7 cells for representative results).
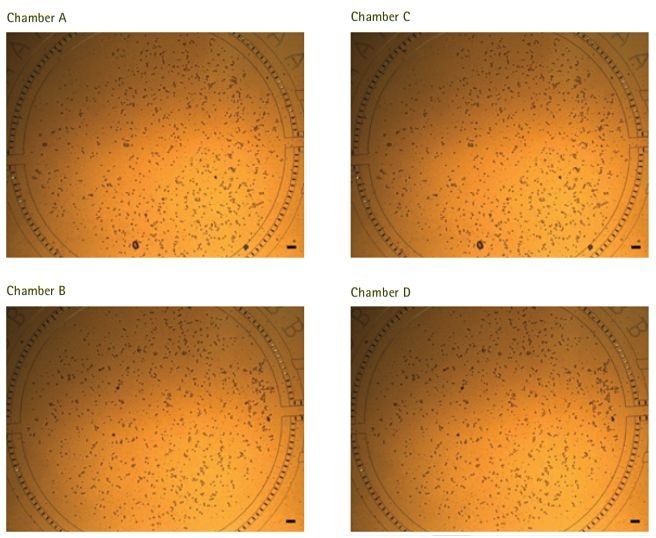
Figure 4. Equal, even pressuredriven cell loading. All four cell chambers of a M04S plate were primed with 0.1% gelatin solution, via capillary action, prior to cell loading. Suspensions of MCF7 cells were prepared at 1.0 x 10 6 cells/mL (for chambers A and B) or at 2.0 x 10 6 cells/mL (for chambers C and D). Inlet well 6 was set to flow at 0.25 psi for 0.1 min (step 1 of the entire protocol as shown in Figure 2) for pressure-driven cell loading.
Long-term cell culture: comparison of automated perfusion culture to traditional, static culture
We compared long-term perfusion cultures of NIH 3T3 cells (144 h), MCF7 cells (168 h) and PC-3 cells (144 h) with static, incubator cultures of these same cells on Millicell® EZ SLIDE. For all three cell lines, cells showed similar morphology and qualitative proliferation rate for both conditions (MCF7 cells shown as representative line, Figure 5). Live/dead staining of MCF7 cells showed very good viability on both culture platforms after 168 hours of culture (Figure 6).
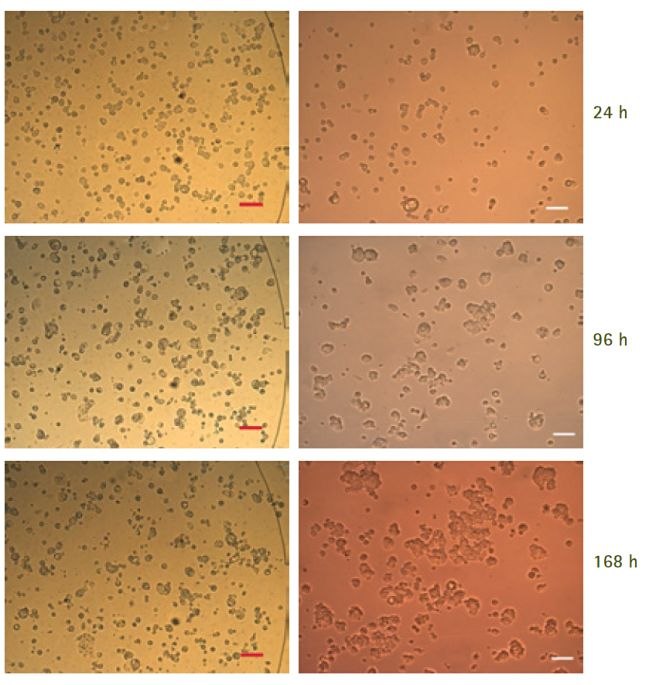
Figure 5. Long-term cell culture of MCF7 cells in the CellASIC® ONIX Microfluidic Platform (left) compared to static culture in the Millicell® EZ SLIDE (right). Even after 168 hours of culture, cells looked qualitatively healthy and showed similar proliferation rates under both culture conditions.
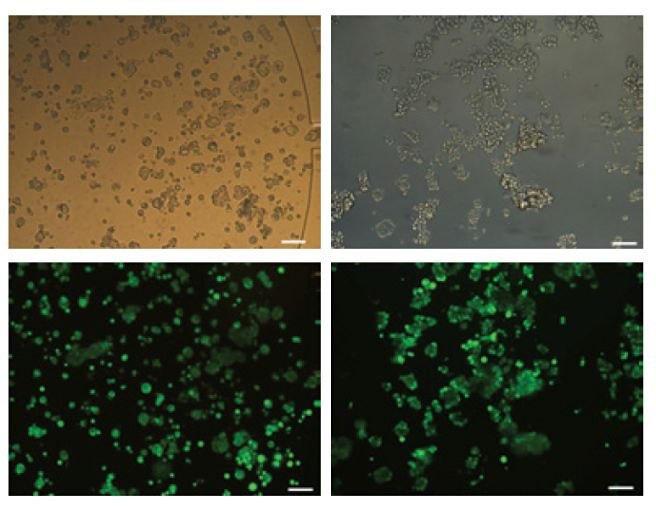
Figure 6. Live/dead staining of long-term cultures of MCF7 cells in the CellASIC® ONIX Microfluidic Platform (left) compared to static culture in the Millicell® EZ SLIDE (right). Even after 168 hours of culture, cells showed high viability under both culture conditions.
Immunocytochemistry (ICC) workflow is facilitated by the CellASIC® ONIX Microfluidic Platform
We created an automated immunostaining protocol (Figure 3) that greatly decreased the hands-on time traditionally required for immunostaining. As shown in Table 3, in-plate immunostaining required 44% fewer hands-on steps than traditional immunostaining.
Weight activity (U/mg)=(U/ml)×1/C |
---|
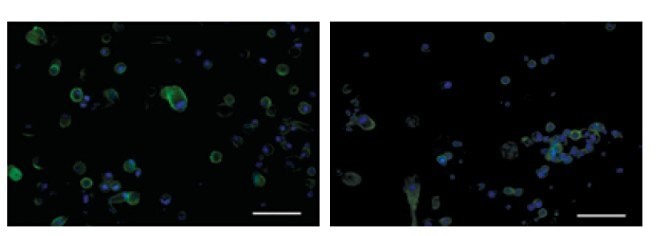
Figure 7. ICC for CD9, a cell surface glycoprotein, on PC-3 cells following a fixation protocol. Both in-plate staining using the CellASIC® ONIX Microfluidic Platform (left) and the Millicell® EZ SLIDE (right) show similar staining patterns.
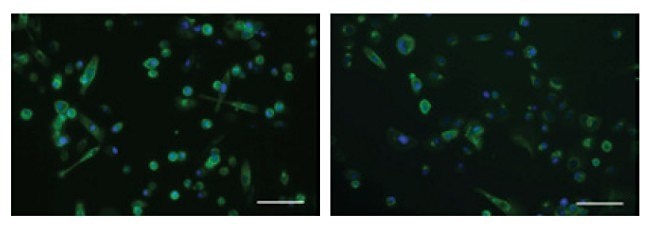
Figure 8. ICC for cytokeratin 19, an intracellular intermediate filament protein, in PC-3 cells following a fixation/permeabilization protocol. Both in-plate staining using the CellASIC® ONIX Microfluidic Platform (left) and the Millicell® EZ SLIDE (right) show similar staining patterns.
Conclusions
We have shown that is possible to maintain a virtually hands-free, healthy cell culture for many days using perfusion feeding: cell cultures in CellASIC® ONIX M04S Microfluidic Plates were healthy, proliferated well and exhibited similar morphology and viability to parallel cultures kept under static conditions in a traditional incubator.
The perfusion conditions we have presented here are the optimized conditions that we obtained by testing different cell lines with varying flow rates, durations, etc. Given our observations, we recommend that users optimize these conditions for their own cells and experimental perturbations.
Specific observations include:
- Pressures between 0.25 to 1 psi were optimal for all three cell types tested. These corresponded to lower flow rates, providing adequate nourishment with minimal mechanical stress to cells.
- Some cell types like the MCF-7 and PC-3 needed some ECM coating to facilitate cell adhesion.
- Optimal initial cell concentration depends on cell types and duration of culture. Here, we used concentrations of 1 x 10 6 and 2 x 10 6 cells/mL for long-term cell culture.
- Loading conditions may need optimization, depending on cell type and cell concentration used.
- Periodically, during long-term culture, waste cells need to be emptied and solution wells need to be replenished. These steps are easily done, because the automated perfusion program can be paused and resumed at any time.
In addition to long-term culture, automated immunostaining is also facilitated by the CellASIC® ONIX platform, for both extracellular (requiring fixation) and intracellular proteins (requiring both fixation and permeabilization). The same concentrations of antibodies were used for both the microfluidic plate and chambered slide, and similar staining patterns were observed in both analyses.
To continue reading please sign in or create an account.
Don't Have An Account?