Trifunctional Probe Building Blocks
Introduction
Chemical probes that mimic a pharmacophore, ligand, or lead compound are of increasing interest for target identification and validation, protein profiling, imaging, or other applications in chemical biology. However, synthesis of the ideal probe is not trivial as even slight structural alterations may have unpredictable effects on target binding and selectivity.1 The synthesis and screening of several analogs is thus often necessary to optimize the outcome of an assay.
In partnership with Pfizer chemists, we have compiled a collection of trifunctional building blocks to enable development of probes. Each possesses three components:
- A connectivity group – NH2, CO2H, OH, CHO, Br, or I – facilitates coupling to ligands.
- A reactive group forms a covalent bond with the protein of interest (POI), allowing even weak-binding events to be captured. Options include diazirines2 and benzophenones3 for UV-activated photoaffinity labeling4 or sulfonyl fluorides for context-specific labeling.5,6
- An alkyne bioorthogonal handle permits click-chemistry conjugation of various azide-containing tags for biotin-streptavidin enrichment or fluorescence imaging.
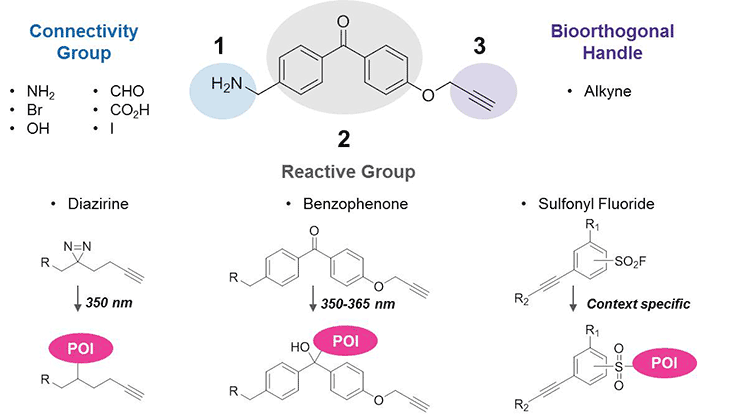
Figure 1.Trifunctional Building Blocks
Advantages
Trifunctional probe building blocks service synthetic chemical biologists in two key ways.
- Reactive groups and bioorthogonal handles are incorporated in one synthetic step.
- Similar connectivity groups enable small library generation.
Applications
These trifunctional scaffolds have been valuable in several applications requiring probe development, such as the examples below.1-3, 6-12 To access these probes, a suggested building block is listed next to each structure.
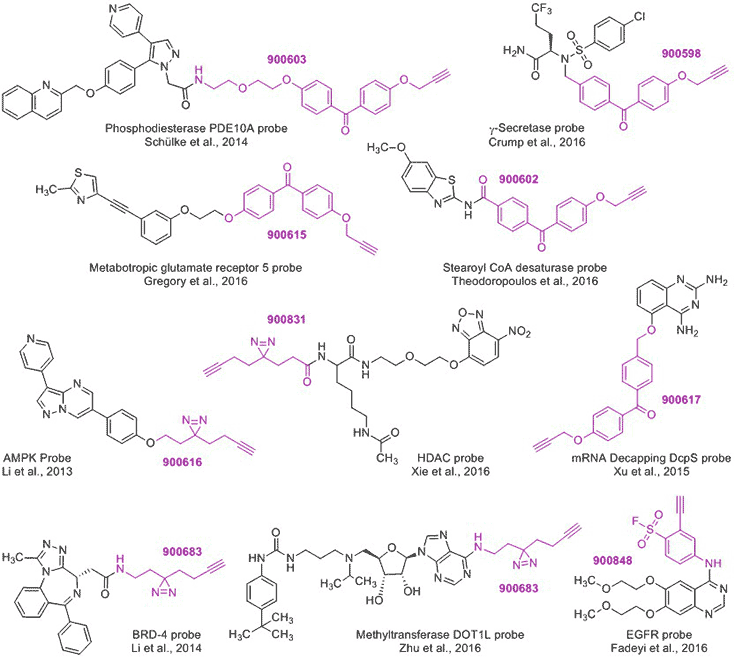
Figure 2.Trifunctional Scaffolds
Library Generation
Similar connectivity groups streamline the functionalization of one bioactive small molecule into a library of potential probes.1 For instance, by using the CO2H-containing building blocks with a ligand’s NH2, a panel of seven probe analogs can be accessed.
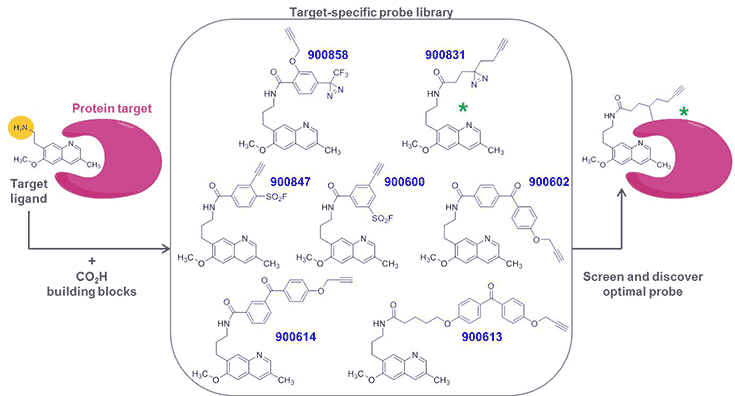
Figure 3.Target-specific Probe Library
Product Offering

Figure 4.Benzophenones, Diazirines, and Sulfonyl Fluorides
References
To continue reading please sign in or create an account.
Don't Have An Account?