Applications of Glucose Polymers in the Synthesis of Diagnostic and Drug Delivery Nanoparticles
Ernest V. Groman, Dmitri Simberg, Guankui Wang
University of Colorado Anschutz Medical Campus
Material Matters, 2019, 14.3
- Introduction
- Dextran Colloids
- Cyclodextrin-Based Nanoparticles
- Illustration of Cyclodextrin NPs from the Patent Literature
- Illustration of Cyclodextrin NPs from Peer Reviewed Literature
- Summary
Introduction
Dextran is a polysaccharide made of many glucose molecules that was first isolated by Louis Pasteur in 1861.1 Since then, dextran has found many applications in medicine including reducing blood viscosity, preventing blood clot formation, and serving as an iron-dextran nanoparticle (NP) to treat iron deficiency anemia using the carboxymethyl dextran-coated magnetite nanoparticle, Feraheme®. Clinical studies have shown that diethylaminoethyl (DEAE)-dextran can reduce cholesterol and triglyceride levels.2 Research applications of dextran include its use as an alternative for PEGylation and as a stabilizer of protein structure.3
Glucose is a deceptively simple molecule until we consider its use as a building block of homopolymers. Glucans are polysaccharides derived from glucose monomers. The monomers are linked by glycosidic bonds. Four types of glucose-based polysaccharides are possible: 1,6- (starch), 1,4- (cellulose), 1,3- (laminarin), and 1,2-bonded glucans. Theoretically, since the anomeric C1 carbon of glucose can have an up or down configuration, we can multiply the 4 listed glucans by 2. Further, cyclic polyglucose compounds called cyclodextrins add to the list of glucans.
In this short review we first examine the role of dextran in forming NPs with doxorubicin and in the formation and stability of iron oxide NPs. In the second part of this review, we discuss cyclodextrin as a starting material for the preparation of NPs. In these reviews we provide methods for synthesis.
Dextran colloids
Dextran is widely used in the synthesis of iron oxide NPs. However, the effect of small changes in the structure of dextran upon the final colloid is not widely recognized. For instance, in an earlier article, Paul et al., found that small changes in the structure of dextran produced profound results in the in the synthesis of dextran-coated NPs.4 The reduction of the terminal reducing sugar had a significant effect on particle size, coating stability, and magnetic properties. For low-molecular-weight dextran molecules (MW ≤10 kDa), reduction resulted in a 10-fold or greater decrease in the carbohydrate-to-iron ratio needed to produce the desired particle size (<20 nm). Particles prepared at the equivalent dextran-to-iron ratio using the equivalent native dextran yielded larger particles. Furthermore, the stability of particle size and coating using 10 kDa native and reduced dextran yielded a colloid that was stable to autoclaving.
Reduced Dextran T10. Dextran T10 (10 g) was dissolved in 100 mL of deionized water at 25 °C to which 1 g of sodium borohydride was added, and the mixture was stirred 12 h. The pH was brought to 5.0 using 6 M HCl. The mixture was ultrafiltered against a 3 kDa membrane. The product was lyophilized to produce a white solid in 63% yield. HPLC retention times (min): reduced dextran) 21.6; native dextran) 21.1.
Preparation of Native and Reduced Dextran T10- Coated Particles. Native or reduced dextran T10 (2.7 g) was dissolved in 70 mL of deionized water. A mixture of 2.0 g of ferric chloride hexahydrate and 1.0 g of ferrous chloride tetrahydrate dissolved in 27 g of deionized water was added. The mixture was purged with nitrogen for 30 min and cooled to 5 °C, and 8.5 g of ammonium hydroxide (28%) added with stirring over 2 min. The mixture was heated at 80 °C for 2 h and purified through six cycles of ultrafiltration against deionized water using a 100 kDa membrane. After ultrafiltration, the reduced dextran particles were 0.2 μm filtered and stored at 4 °C.
Feraheme (ferumoxytol) is one of the few dextran-coated NPs that has been approved by the FDA and is indicated for treating anemia. The iron in Feraheme is found in the form of magnetite encapsulated in a dextran coat. The dextran that covers the magnetic core of Feraheme is both reduced and modified with carboxymethyl groups, providing the technological advance that contributed to its approval by the FDA.
The exact details of the synthesis of Feraheme is a trade secret. However, Feraheme is protected by US patents 6599498, 7048907, 7553479, 8501158, 8926947, and 9555133. For those who are interested, a close reading of these patents can provide an understanding of the art and science of making dextrancoated colloids.
Besides iron supplement application, Feraheme has also been widely used as a T2 contrast agent for magnetic resonance imaging (MRI) in both basic research and clinical trials. After intravenous injection, Feraheme is internalized by macrophages. While Kupffer cells in the liver are the primary means of internalization, other tissues such as spleen, lymph nodes, or tumor sites have also been shown to internalize Feraheme.5 Studies have identified Feraheme as a potential diagnostic contrast agent to detect inflammation sites and tumors, monitor treatment after brain surgery and map out metastatic lymph nodes for certain cancers using MRI.6
Wasiak et al. present an alternative method for synthesis of dextran-based colloids using multiple steps beginning with the oxidation of dextran yielding multiple aldehyde groups.7 In their example the aldehydes are reacted with doxorubicin and hydrophobic amines referred to as coiling agents. The procedure is described below.
Synthesis of polyaldehydodextran (PAD). The dextran (10 g) was dissolved in 200 ml distilled water and sodium periodate followed by the addition of sodium metaperiodate. After stirring in the dark at room temperature for 1 h ethylene glycol was added to quench the oxidation by periodate. The solution was dialyzed against distilled water and dried at 50 °C.
Synthesis of dextran nanoparticles (Dex-NPs) and with doxorubicin (Dox-NPs). Dried PAD (1 g) was dissolved in 10 ml of water (30 °C) and combined with 0.01 g/ml of doxorubicin aqueous solution. Five minutes later coiling agent (hexylamine, octylamine, dodecylamine, or benzylamine) was added, and the mixture was stirred for 30 min at 30 °C. The pH was increased to pH 10 with 0.5 M sodium hydroxide within 60 min. The pH of the mixture was decreased to 7.4 and the Dex-NPs were dialyzed against water. Dex-NPs were lyophilized using dextran as a cryoprotectant. The schematic representation of the NP synthesis is presented on Figure 1A.
Synthesis of dextran nanoparticles with reduced bonds (rDox-NPs). Bonds between dextran aldehyde groups and amine groups of doxorubicin were reduced with NaBH4. The pH of the mixture was brought to 7.4 and rDox-NPs were dialyzed against distilled water for 24 h (Figure 1B). Reduction binds doxorubicin covalently to form rDox-NPs. The rDox-NPs were used for the calculation of the drug encapsulation efficiency.
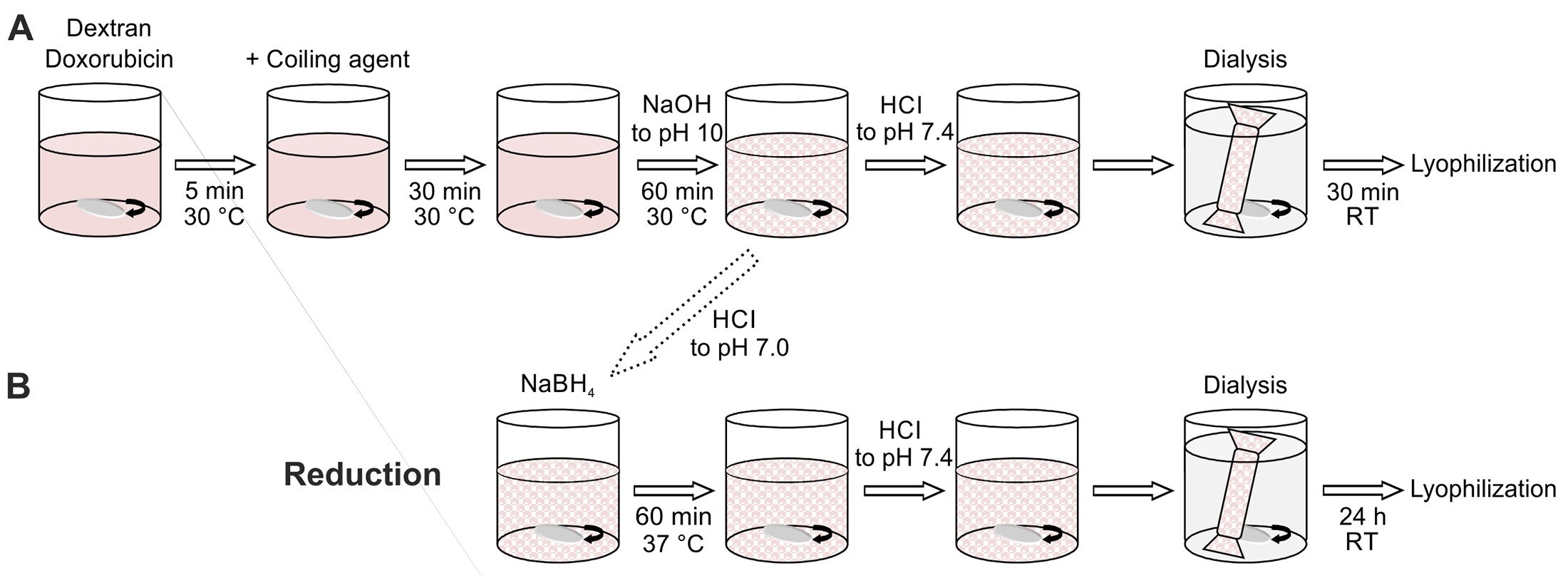
Figure 1. Diagram of Nanoparticle Synthesis Strategy using Dextran.
Cyclodextrin-based nanoparticles
Cyclodextrins consist of a macrocyclic ring of glucose subunits joined by α-1,4 glycosidic bonds (Figure 2A). They find applications in food preparation, pharmaceutical formulation, and drug delivery as well as chemical, industrial, agricultural, and environmental applications.3 Cyclodextrins are known to the general public since they are commonly used in products designed to control odors in the home. Cyclodextrins can be expensive, but industrial and consumer usage has reduced prices to a range acceptable to academic laboratories. Cyclodextrin are all considered GRAS, or generally recognized as safe, by the U.S. FDA.8
Cyclodextrins bind hydrophobic molecules in the center of the ring (Figure 2B). With a hydrophobic interior and hydrophilic exterior, cyclodextrins form complexes with hydrophobic compounds conferring solubility and stability to hydrophobic compounds. Cyclodextrins form inclusion compounds with hydrophobic molecules, enabling them to penetrate body tissues. The mechanism of ligand release is related to a variety of factors including pH, ionic strength, solvent polarity, temperature, and enzymatic degradation of the ligand and/or cyclodextrin.3
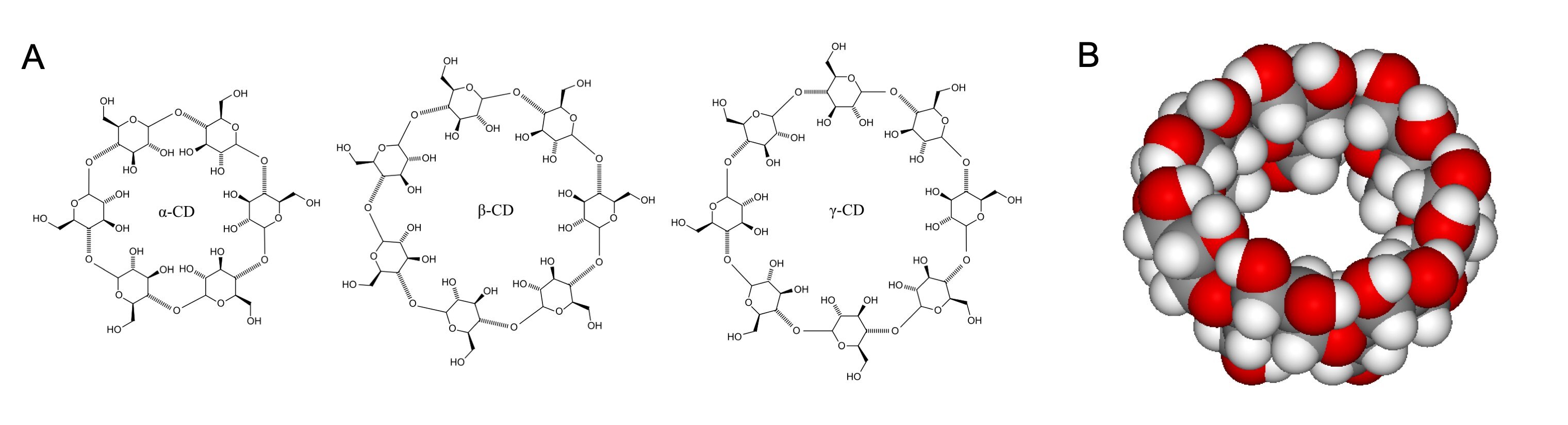
Figure 2. A) The three most common cyclodextrins; B) a space-filling model of β-cyclodextrin.
Illustration of cyclodextrin NPs from the patent literature
The affinity/avidity constant between cyclodextrin and bound ligand can be increased considerably by polymerizing cyclodextrin into an NP. This section considers several approaches to the polymerization of cyclodextrins based on the patent and peer-reviewed literature. Examples of forming cyclodextrin NPs from the patent literature are noted for their simplicity and scale, reflecting industrial applications.
Water-insoluble cyclodextrin polymers are produced by polymerizing cyclodextrins with crosslinking agents. Generally, epichlorohydrin or diepoxy derivatives (e.g. diepoxy butane, diepoxypropyl ether, ethylene glycol diepoxypropyl ether) are used as cross-linking agents, with the polymer being produced either by block polymerization (U.S. Patent 3472835), as regular pearls (GB patent 1244990) or as foamed polymerizates (Hungarian patent application 1188/81). The mechanical properties of these rigid materials can be improved significantly by incorporating polyvinyl alcohol (U.S. Patent 4274985) with the crosslinking reagent. This is important when using cyclodextrin polymers as stationary phase in column chromatography.
When using cyclodextrin polymers as stationary phase in chromatography, important attributes are related to the waterabsorbing capacity and rate of water absorption. The waterabsorbing capacity of the final product is greater when more cross-linking agents or more dilute cyclodextrin solutions are used. An example of the synthesis of a rapid swelling cyclodextrin polymer is found in US Patent 4547572.
In 10 ml of water (60 °C), dissolve 3.0 g NaOH, 5.0 g β-cyclodextrin and then add 2.5 ml of tetraethylene glycol and 5.7 ml of epichlorohydrin. Continue heating for 1.5 hours at 60 °C. The cooled gel is washed salt-free with water, dehydrated with acetone, dried at 105 °C and pulverized. After this process is complete, 6.8 g of a white powdery material are obtained with a cyclodextrin content of 50.6%.
It is desirable to form beads to facilitate flow during chromatography. One example (U.S. Patent 4726905) uses polycyclodextrin beads to remove polychlorinated biphenyl compounds from the environment. US Patent 5075432 illustrates an improved method for forming cyclodextrin polymer beads.
In a 3-liter reaction vessel equipped with a stirrer blade, add 2 liters (1,540 g) of methyl isobutyl ketone (MIBK) and heat to 80 °C. The top of the reaction vessel is covered with a condenser and the stirring blade is run at 300 rpm. To this reaction vessel, add 6 g of polyvinyl butyral (hydroxyl content of about 19% and a molecular weight of about 125,000) and stir until dissolved. In another vessel, a slurry is prepared by mixing 49 g of β-cyclodextrin, 54.5 g of water and 87.3 g of a 30% NaOH solution. The stirring is continued until the solution is murky but transparent. Then, the cyclodextrin slurry is added to the reaction vessel containing the MIBK and polyvinyl butyral. After 5 minutes of stirring, add 136 g of epichlorohydrin and continue stirring at 300 rpm. During the reaction, the temperature is maintained to 80 °C. After 4 hours the particles are recovered and washed. The particle diameter ranged from 30 to 170 nm.
Our last example from the patent literature of forming cyclodextrin polymers (US Patent 7745558) illustrates that polymerizations can be performed at room temperature in contrast to the previous two examples where polymerization occurred at 80 °C.
β-cyclodextrin (2.5 g) is dissolved in a NaOH solution (2.5 g / 7.5 ml). Epichlorohydrin (4.4 ml) is gradually added to the solution over 20 minutes. The mixture is stirred at room temperature for 4 hours. The resultant polymer in a gel state is immersed in a 0.3 M CaCl2 solution to be cured. The cured polymer is washed with water and ethanol, dried at 70 °C and pulverized. β-cyclodextrin polymers have an average particle diameter of 200 nm.
Illustration of cyclodextrin NPs from peer reviewed literature
Please note that for the two peer reviewed reports presented below, the scale and complexity of synthesis are considerably smaller and more complex, respectively, than that found in the patent literature. The sheer complexity of peer reviewed papers in this discipline is reflected in the number of authors and laboratories involved in a single manuscript, indicating the new reality that a variety of technical specialists are required to accomplish particular tasks and resulting in funding agencies expressing interest in supporting collaboration.
In our first peer reviewed example, Kim et al. sought to improve the detection of tumor-associated macrophages (TAMs), which are widely implicated in cancer progression.9 Since it is difficult to quantify total TAM numbers and their dynamic distribution in a non-invasive manner, they developed a 64Cu-labeled polyglucose NP for PET imaging which they named Macrin. A simplified summary of their very complex synthesis is presented below and illustrated in Figure 3.
Macrin-NP. Carboxymethylated polyglucose was activated with EDC and NHS in MES buffer. L-Lysine was added. The reaction mixture was added to ethanol and a white pellet was collected. The pellet was dissolved in H2O, filtered dialyzed. Macrin nanoparticle (Macrin-NP) was filtered and lyophilized to give offwhite solid particles.
NODA-GA-Macrin. Macrin-NP was dissolved in H2O, mixed with NODA-GA-NHS and reacted with succinic anhydride. The crude mixture was purified by dialysis and lyophylized. The hydrodynamic diameter of the NODA-GA-Macrin-NP was 20nm. The NODA-GA-Macrin-NP was ready to be charged with 64Cu and drug.
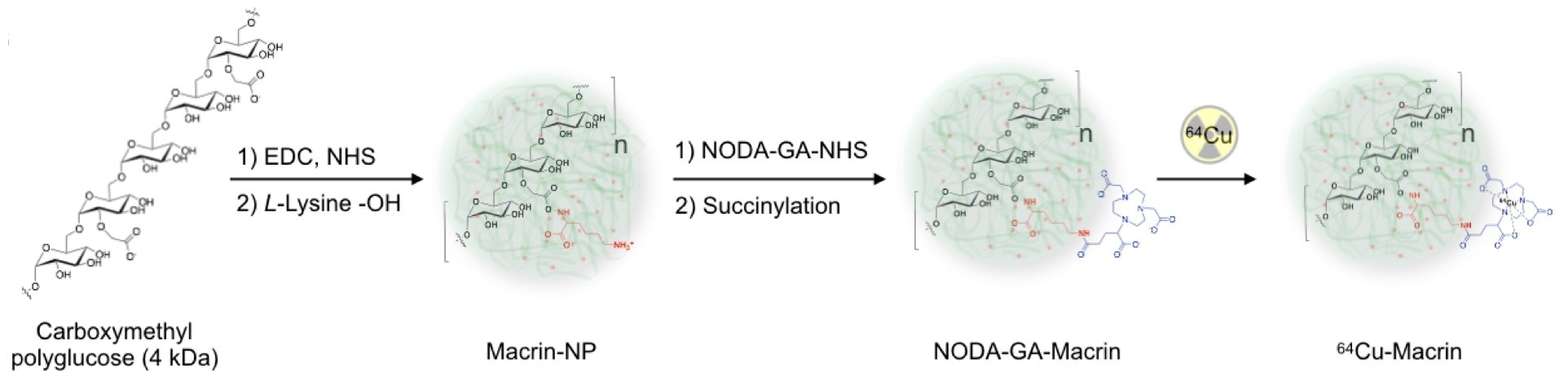
Figure 3. Macrin synthetic scheme.
In another report, cyclodextrin is conjugated with penynyl chloride and then crosslinked with epichlorohydrin in the presence of a 4 µm sized silica particle (Figure 4).10 The suggested use of the micron sized particle is for water purification. The authors suggest introduction of pentynyl ligands improves the capturing efficiency of the hybrid material. The scale of this reaction is 5 grams.
PyβCD. β-cyclodextrin is dissolved in N,N-Dimethylformamide followed by the addition of Li-dimsyl. Pentynyl chloride is added and stirred at room temperature for 24 h (Figure 4). After the solvent is removed, the residue is suspended in water and precipitated in acetone. The product was recrystallized and dried.
Poly-PyβCD. PyβCD (2 g) is added to 40 ml of water followed by the addition of 6.8 ml of the 20% NaOH. The mixture is stirred at 60 °C. Silica particles (2 g) are added, and 162.84 mg of epichlorohydrin is added and stirred at 60 °C. The product is washed and dried. For comparison, β-cyclodextrin and PyβCD were polymerized without silica particles following the same procedure as described above and precipitated in acetone (Figure 4).

Figure 4. Scheme for the synthesis of β-cyclodextrin polymer (Poly-βCD, 1), pentynyl ether of βCD (PyβCD, 2), polymer of PyβCD (Poly-PyβCD, 3), and hybridization with SMP (Poly-SMP- PyβCD, 4).
Summary
In this review, we briefly discuss the applications of two categories of glucose polymers used in the synthesis of nanoparticles: dextran and cyclodextrin. Dextran-coated iron oxide nanoparticles are clinically approved for treating anemia and are being investigated for use in conducting MRI of specific organs and tissues. Both dextran and cyclodextrin polymers have been used to deliver therapeutics and diagnostic agents in either nanoparticle or microparticle forms. These glucose polymers hold great promise for synthesis of novel biomaterials in the pre-clinical and clinical applications.
To continue reading please sign in or create an account.
Don't Have An Account?